Understandably. the British Government had considerable interest in the design, manufacture and performance facts and figures regarding the German rocket cargo ship. The British knew a great deal about what it did when it came down. They even knew quite a bit about how it behaved in flight. Radar—particularly the giant long-range microwave early warning set that worked from the southeast of England—had been able to track the take-off flight, and entire trip of the rockets on their way up. over, and down again. The British-American knowledge of the flight cliaracteristics of the rockets may well have been better than that of the Germans; our radar equipment was markedly more accurate, and had markedly greater range.
The trouble with all this knowledge of the flight of the V-2 was that while we could send our radar pulse's out to hit and return with the desired information, there was no weapon in the Allied armory capable of getting where a V-2 was when the V-2 got there. At the moment the last of the fuel was exhausted, the V-2 had a velocity very near one mile a second: when it was dropping onto England, the velocity had been decreased—by air resistance during the passage—to about half a mile a second. That was still far too fast for any antiaircraft shell.

But the British very much wanted to now what the V-2 was like on take-off. During the defeat of Germany, when Anglo-American armored columns were slicing across Germany faster than German communications could report their progress, a train loaded with V-2's was captured—complete with detailed operation instruction manuals. After the job of conquering Germany was finished, Allied research teams set up and launched a V-2. recording the entire process on movie film. The accompanying photographs are stills taken from that film. If you've seen the film in newsreel form, you saw that the giant rocket rises, at the start, with a strange deliberation, as steady and straight as an elevator. It must be an incrediblc scene; the giant and massive machine, hissing furiously, suspended in midair and rising steadily on the jetting flame.

The third photograph, on page 101 [above], shows clearly the remarkable degree to which the jet-design directs all the exhaust gases in one straight, tight column, with practically no lost—sidewise—motion.

HEADACHES FOR RADARS
No weapon which depends on sensitivity, skill and finesse for it operationi is immune to counter-attack; radar, as the ultimate in sensitivity, skill and finesse, is. inevitably, wide open to countermeasures—and the Allies, who had the best radar equipment, had the best countermeasures. Actually, the indications are that radar is a one-war weapon, a weapon of surprise. where "surprise" is interpreted to cover a period of months or a few years. Actually, the Germans succeeded in countering our early radar sets very quickly; the Allied radar was able to continue as a highly effective weapon only because the continuous development of new techniques, new wave-bands, proceeded faster than the German laboratories could follow. To quote Kipling:
"They copied all they could follow.
"But they couldn't follow my mind—
"So I left 'em, swcatin' and stealin',
"A year and a half behind."
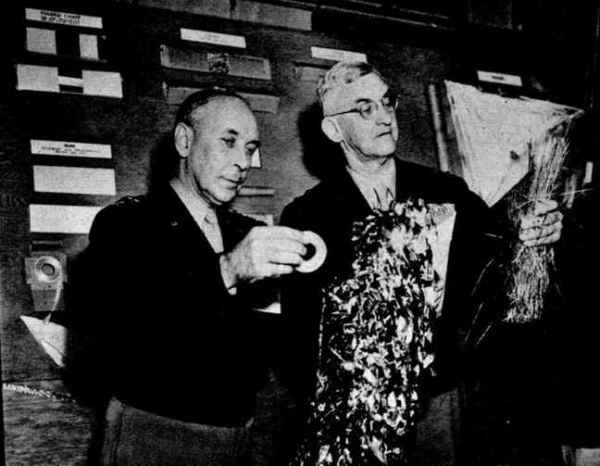
In radar. countermeasures, the American developments were enormously advanced. First, simplest, and one of the most effective was the use of aluminum foil strips—the material shown on page 103 [above]. Radar operates by transmitting a sharp pulse, and then waiting in complete silence for the echo, and very accurately timing that echo. The strength of the echo indicates, normally, how large an object was struck.
But radar frequencies are astonishingly tike sound frequencies. The wave lengths are about the same, and the waves can set up semimechanical resonances in metal. Tuning is done with resonant cavities; wave lengths can be measured directly with callipers by setting up a conductor and varying its length until electrical resonance occurs, and then simply measuring that length. Aluminum foil, cut to that determined length, will resonate—"ring"—when struck by the radar wave, for a considerable time—on radar's time-scale—after being struck, and return an "echo" that looks millions of times stronger than the echo from such a flimsy thing has any normal right to. One piece of aluminum foil can appear, on a radar scope, as large as a whole nonresonating bomber. That makes for confusion.
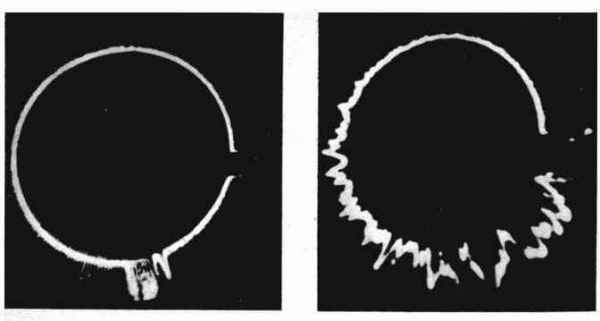
On page 104 [above] is a picture of that confusion; at the left, a German radar scope with a plane spotted; at right the same scope after the plane has dropped a few bunches of aluminum foil. The photographs were taken front a German training film: the Nazis didn't like the foil at all.

On page 102 [above] is a picture of a far more complex method of balling up the works: a radar jamming transmitter. It sends pulses too. It is very carefully tuned to the enemy radar frequency, and to the enemy radar's pulse-rate. But the pulses it sends are, of course, quite meaningless, and when a collection of jammer-equipped planes flies overhead, the data furnished by the radar below are as intelligible as the gibberings of a lunatic.
America's final triumph, though, can't be shown as yet. It was known as "Tuba," technically as a "resnatron," and generated a stupendous, and stupefying flood of radar energy—sheer, terrific noise. Two hundred miles away its blatting blasts of radar noise completely bollixed the Luftwaffe's night-fighter radar. It was like trying to hear the echo from the cliff across the way white a man on the cliff delivered a continuous, very loud Bronx cheer. Tuba, in England, defeated Cierman radar over Germany.
Though probably useless henceforth as a prime war weapon, the microwave techniques developed for radar have new uses. Page 105 shows the "lamp" and reflectors for microwave spotlights. These microwave projectors throw sharp beams that can be used for commercial communication transmission, doing away with the need for wire links in crosscountry communication networks. China, without telephone links today. will probably install such service, with the result that, ten years hence, China's telephone system may he more modern than America's!
Radar, as everyone knows, was purely an electronic problem. Sadly, it wasn't—not by a long, long way. It was also an aerodynamic, plastic, metallurgical, machine-tool, and. in fact, general industrial problem.
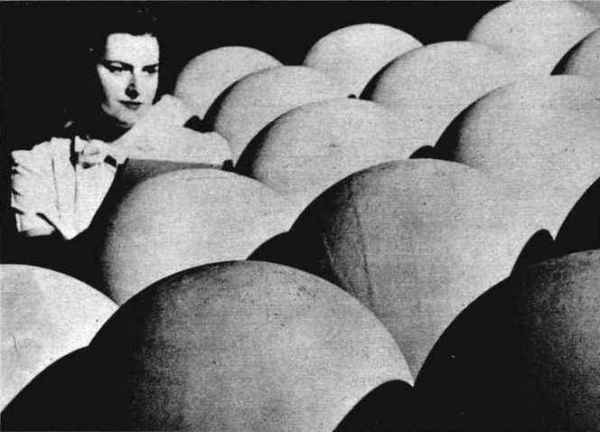
Above are a collection of radomes—domes for radar antennas. A radar projector, by the nature of things, involves a parabolic reflector. or something equivalent; in no case is it even remotely conducive to streamline form. If carried on a plane, it has to be enclosed in a streamlined shell, or dome—like these.
But then comes the headache. The dome must be perfectly transparent—to radar—and must not refract the radar beams appreciably. Oh, yes: radar beams can be refracted—Hertz demonstrated that. The dome obviously can't be metal, so it must be plastic.
But not just any i>lastic. To radar, standard bakelite is like heavily darkened glass is to light. Polystyrene is the ideal plastic for high-frequency radar—it's almost jjerfectly transparent. Unfortunately it isn't too strong, and. worse, melts at a low temperature—about like a hard wax. With a really fast modern plane, air-friction, and air-compression causes very severe heating, more than enough to melt polystyrene; enough to damage even resistant phenol-formaldehyde plastics in certain locations on the ultrafast planes. VVestinghouse made these: the composition is not revealed!

The modern roc lays a super-modern egg, the radio-operated controllable bomb. The "high angle" refers to the fact that, since this bomb does not have wings, it has a gliding angle somewhat steeper than that of the traditional kitchen sink. The radio controls can't keep it from falling, but by tilting the angle of the airfoil section band around the bomb, the direction of the fall can be controlled to a considerable extent.
In high-altitude bombing, it is possible to make proper allowance for plane speed, direction and altitude. the plane's air speed vs. ground speed, the rate of fall of the bomb, and the effects of gravity and air resistance. But you cannot take into account all the varying lower-level winds, because they are necessarily unknowns. Hitting a pin-point target, such as a railroad bridge, from high altitude beconies a matter of chance.
The ROC's egg. on the other hand, can set up its own local "'winds" of sufficient strength to blow it Itack onto the course, and assure a dead-center hit.
The Nazis tried to develop something of the sort, but their dirigible bomb was controlled by electric signals carried by a couple of miles of fine wire that the dropping bomb spun out behind it as it left the plane, like a spider dropping on her thread.

THE BETATRON
Just before the United States got into the war. Astounding reported the invention of the betatron. It was mentioned in one story—"Recoil," by George O. Smith—but the betatron, like atomic fission, has been under wraps since. This is one of the first pictures released.
Built by the Gencral Electric Labratories. the betatron is a trick super-transformer. Theoretically, a transformer can convert any voltage of alternating current into any desired voltage—110 volt household power to 110.000.000 volt power, for instance. The trouble with this theory is that the electrostatic stress represented by 100.000.000 volts is more than any insulation can endure. Actually. 2.000,000 volts is about the limit for an ordinary transformer system.
The betatron is, nevertheless, a transformer which produces 100.000,000 volts—or to be more accurate, the equivalent thereof. It produces a kinetic energy in electrons equivalent to that the electrons would accumulate in falling through a pote»tial of 100,000, 000 volts.
The huge magnetic core of the betatron resembles that of a cyclotron: The big magnetic coils used resemble those of the cyclotron. But there the resemblance ceases: the magnetic core of the cyclotron is actually made of great, solid slabs of soft iron. The betatron's core is made up of laminations of transformer iron; it is an alternating current, rather than a direct current magnetic device.
The cyclotron uses a magnetic field to hold the fast-moving ions under control: the energy is fed into the ions from a 10 megacycle, 100.000 watt short-wave radio transmitter. In the betatron the magnetic field controls the motion of the electrons, but the magnetic field is itself the source of their energy.
In essence, the betatron is a transformer, the secondary winding of which consists of a vacuum tube—a doughnut shaped vacuum tube around the core of the transformer. Electrous are shot into this tube from a standard electron-emitting filament at the instant that a bank of powerful, high-capacity condensers is discharged into the big magnetic coils. The discharge of the stored energy in the condensers builds up the magnetic field in the coils and the core. The electrons which have been shot into the vacuum-tube doughnut act precisely as the electrons in a copper wire wound around the core would; the increasing magnetic field accelerates them. At the same time it accelerates them, the magnetic field forces them to take curved trajectories— to go around the core in a circular orbit instead of taking off on a tangent.
The rising magnetic field constantlv accelerates them, which tends to make them go in larger orbits because of higher speed. But simultaneously, the increasing strength of the magnetic field tends to force them into narrower orbits. The two effects are mathematically equal and opposite; the electrons remain in a circular orbit.
It takes approximately 1/240th of a second for the condenser bank to discharge into the magnet coils. In that time, the electrons make about 250,000 revolutions around the core, and are accelerated to a speed equivalent to 100.000,000 volts. They are traveling at speed so close to the speed of light that they are nearly l/10th as massive as protons—about two hundred times heavier than at the start.
At the instant the condensers have drained into the coils, a pulse of current applied to an auxiliary coil causes the swarm of electrons to deflect and strike a tungsten target which they had missed previously. X-rays are produced—but X-rays vastly more penetrating than the hardest gamma rays produced by radioactive elements. Only the fission of uranium can equal in violence the smashing blow these super-accelerated electrons deliver.
The enormously high energy level of the X-rays given off has the interesting effect of causing nearly all the energy of the quanta to be ahsorlied in creating electrons and positrons out of empty interatomic space, rather than simply being absorbed as heat in the blocking material.
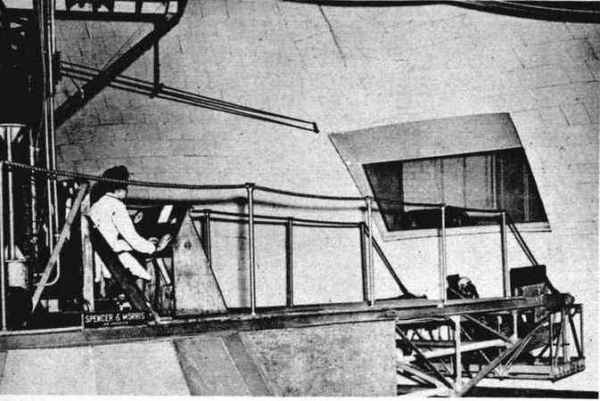
G-STRING TO ANTI-G-SUIT
The one-man merry-go-round above is guaranteed to cause a sensation in anybody, including the most hardened roller-coaster addict. If nothing less suits, it will produce blackout—in fact it was specifically designed to do just that, duplicating in its full unpleasantness the effect of a 9-G pull-out of a dive Itomber. Designing a machine to produce the effect isn't anywhere near as simple as it might seem. Getting the necessary 9-G is easy—the long arm with the gimbal-swung chair at the end takes care of that. But the essential feature of an actual 9-G pull-out is that it doesn't last very long. The trick in designing a machine to test the phenomena involved was to make one that would build up the 9-G effect in a matter of a very few seconds, and then back off the 9-G to a more reasonable level in an equally small number of seconds.
The purpose? To find out how to make the anti-G suit.
Blood may lie thicker than water, but it has the same habit of running downhill when gravity, or other acceleration, pulls. It runs from the brain and eyes, and heart, where it's needed, to the feet, ankles and legs where it is not needed, and not wanted. The blood vessels in the brain and eyes cullapse, the blood volume available for the heart to pump practically vanishes, while the blood vessels of feet and legs and lower abdomen become vastly and dangerously distended.

The anti-G suit was rigged to overcome that. The Germans tried using a suit consisting of an inner waterproof rubber garment and an outer, inelastic garment, the space between being filled with water. Under acceleration, the water tried to go the same way blood did, thus crowding the space in the inelastic outer suit and forcing the blood back where it belonged. Too clumsy—pilots wouldn't wear them.
The University of Southern California helped develop this suit. Infatable air-packs supply counter-pressure in legs and abdomen, and force the blood back toward the head. The amount of squeeze applied by the air-bags is controlled by a small valve regulated by an accelerometer. More Gs, more air.
The suit works—and is comfortable in between dives.


Click to join 3rdReichStudies
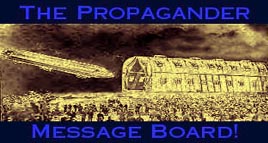
Disclaimer:The Propagander! includes diverse and controversial materials--such as excerpts from the writings of racists and anti-Semites--so that its readers can learn the nature and extent of hate and anti-Semitic discourse. It is our sincere belief that only the informed citizen can prevail over the ignorance of Racialist "thought." Far from approving these writings, The Propagander! condemns racism in all of its forms and manifestations.
Fair Use Notice: The Propagander! may contain copyrighted material the use of which has not always been specifically authorized by the copyright owner. We are making such material available in our efforts to advance understanding of historical, political, human rights, economic, democracy, scientific, environmental, and social justice issues, etc. We believe this constitutes a "fair use" of any such copyrighted material as provided for in section 107 of the US Copyright Law. In accordance with Title 17 U.S.C. Section 107, the material on this site is distributed without profit to those who have expressed a prior interest in receiving the included information for research and educational purposes. If you wish to use copyrighted material from this site for purposes of your own that go beyond 'fair use', you must obtain permission from the copyright owner.
Sources: Two superior Yahoo! Groups, magazinearchive and pulpscans, are the original sources for the scans on this page. These Groups have a number of members who generously scan and post rare and scarce magazine and pulp scans for no personal gain. They do so for love of the material and to advance appreciation for these valuable resources. These Groups are accepting new members; join now!