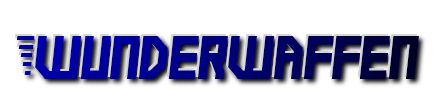
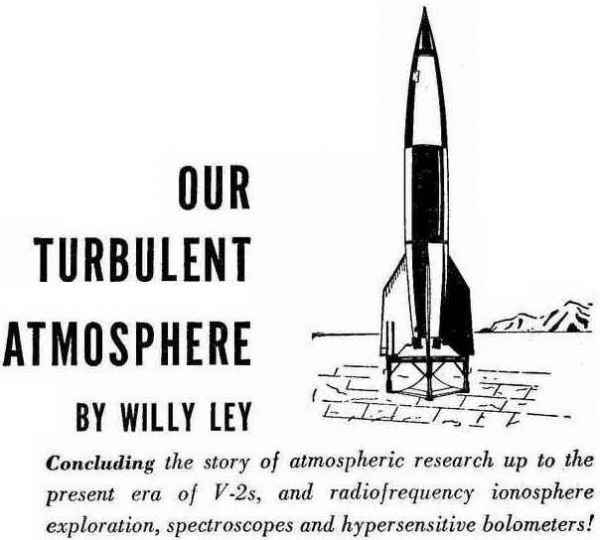
Part 2
I mentioned that American engineers tied small transmitters to balloons in the hope of following their flight in spite of darkness or haze or clouds. Just what happened—or didn't happen—on the French battlefields is not known, presumably the information was secret at first and later, after it was declassified, nobody bothered to write it up. In 1921 a German engineer by the name of Herath released balloon-borne spark transmitters but also without success. The signals could be picked up only over such short ranges that it was not worth while; confirming the scoffing of those who maintained that a spark transmitter's acoustical range was greater than its electrical. But two years after Herath, W. R. Blair of the United States Signal Corps repeated the experiment and succeeded in tracking a balloon for twenty minutes and to an altitude of twenty thousand feet.
It could he done, as was also confirmed by experiments in France, but it didn't mean much. The transmitter could only say "I am here!" but could not tell anything else yet. The idea was to take one of those instrument sets as had been originally developed by Assmann, Besancon and Hermite and make a transmitter broadcast their readings. And suddenly the news came that Professor Pyotr A. Moltchanoff of Slutsk in Siberia had done just that, he had succeeded in transmitting temperature and pressure readings from an unmanned balloon. It wns a genuine Russian "first" and it was the beginning of what is now called "telemetering" but should be called "radio-telemetering". Moltchanoff was the first to accomplish radio-telemetering, telemetering over a wire had been started in 1877 by the Dutch instrument maker Olland of Utrecht.
Lecture experience has taught me that telemetering of any kind is regarded as such advanced magic bv many people that they don't even ask how it is done, feeling sure that only the initiated could possibly understand it. For this reason I'll explain Olland's method with the aid of a factory clock which shows hours and minutes and also seconds bv means of a sweep hand. Supposing you have a seashore station which is to inform you about the rise of the tide and about the direction of the wind. Now we take our factory clock and gear a float to the long or minute hand, while a weather vane is geared to the short or hour hand. The sweep hand we leave alone to travel round the dial once every sixty seconds. Every time it passes over the "12" it makes a short contact. Every time it passes over the other hands it makes a contact too, the contact for one of the two hands lasting a little longer than that for the other. Each contact sends an electric impulse through a wire, and at the other end of the wire you get a short impulse at regular intervals from the "12", which is called the "reference pulse" while the other two supply the actual readings.
It was a modification of this system which Moltchanoff used, except that the wire was replaced by a carrier wave. A lot of such systems were thought up and tried, and some actually used, during the interval from 1930 to 1940. One, invented bv a Finnish scientist, Dr. Vilho Yaisala, transmitted readings by changing the wave length of the carrier wave. The most customary American system is the so-called Diamond-Hinman circuit which operates with modulations of the carrier wave. One of the most recent ones uses a record with concentric grooves—riot a spiral groove as in ordinary records—each groove carrying letter combinations in Morse code. The sensing instruments have arms which reach into those grooves and broadcast their reading bv the groove they picked. There are three sensing instruments, for air pressure, temperature and humidity and they jab at the record in sequence with an interruption for reference, like this: pressure, temperature, humidity, pause, pressure, temperature, humidity, pause, and so on, until something or somebody gets tired. All this goes over the same carrier wave, but you can imagine that you have a lot of readings to take so that you have to add "
Very ingenious instruments indeed, but something has to carry them upstairs. Airplanes were used when convenient, but the bulk of the carrying of such radio sondes was done by small rubber balloons which expanded as they rose. Finally they burst and the package of instruments came back by parachute, not so much for the sake of recovery, which was never very good, but in order to avoid damage claims. Most of the carrying balloons burst between fifteen and twenty kilometers only rarely did one go higher. People who advocated rockets as instrument carriers for the sake of higher altitudes—and also because a rocket does not get stuck at a low altitude because there happen to be icing conditions around—were usually informed by meteorologists that they did uot care much since conditions in the stratosphere did not influence conditions in the troposphere. This is almost certainly wrong, although you can probably do without stratosphere conditions for an ordinary weather forecast.
Suddenly the V-2 was available, instruments could be carried to one hundred fifty kilometers or better and now we are really beginning to learn something about the high atmosphere. The balloons just rose above the tropopause, the V-2 goes considerably beyond the stratopause. Because newspaper releases cannot be too technical most of the public thinks that these rockets carry instruments in their war heads only. Actually, when you look at a V-2 ready for a stab into the ionosphere you see—or could see if the metal skin were transparent—research instruments stuck into every bit of empty space. Most of them are in the war head, of course, but some were located in the compartment below the war head which houses the rocket's own guiding instruments. Some could be crammed into the main section where there was a small space between the two fuel tanks. Others were fitted into the hollow stabilizing fins. As for antennae, they were sometimes strung from the stabilizing fins to a point higher up on the rocket, or else trailing antennae were used which the rocket pulled out of their boxes when it rose.
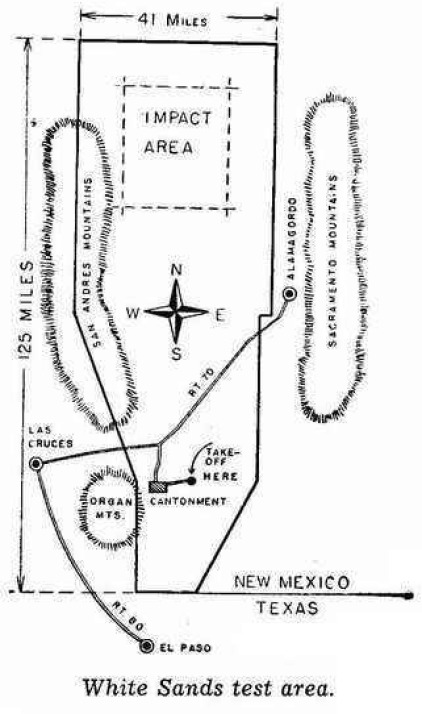
One major difficulty was presented by the simple fact that a V-2, coming back from a high altitude trajectory, does about as much damage with a war head full of cosmic ray counters as it would with a war head full of TNT. Of course nobody cares about a sixty-foot crater in the New Mexico desert but the sad fact was that the rocket itself formed part of the debris, in very small and useless fused pieces. And while most of the information gathered by the instruments can be telemetered some things just have to be recovered. Film, for example, whether it took a picture of the horizon or of alpha particles. And seeds which have been carried to high altitudes to be exposed temporarily to the conditions of near-empty space have to be found and planted if the experiment is to be of any use. A parachute for the whole rocket was out of the question. One could probably build a parachute which can carry four tons, the returning weight, but it probably would not fit into the available space, even if all the space were turned over to the parachute.
To supply some instruments with small individual parachutes is an obvious thought and it was tried, too. So far the outcome is doubtful, sometimes an observer claimed to have seen a floating parachute but then it was not found and one can't be sure whether the observer might not have been mistaken. And even if he did see a floating parachute it is by no means certain that it also carried its instrument, it might have torn off instead of pulling the instrument out.
Pondering the problem somebody found the answer. The rocket buried itself in the desert in a deep impact crater because it struck the ground nose first like a bomb. It struck with a high velocity because it is nicely streamlined—up to the moment of impact—and the stabilizing fins keep the tail up. In short the empty rocket had nice ballistic characteristics, after all it was designed with that end in view. Destroy these characteristics and you'll get much less ot an impact. If, for example, you broke the war head off the falling rocket that war head, by itself, would probably tumble through the air and strike the ground at an odd angle. The after body ought to do even better. It would not have a nose at all, but a jagged hole in front. The stabilizing fins would try to straighten it out while falling, of course, but they would be handicapped by having the heaviest component left, the motor and the turbine-pump aggregate, at the wrong end. The after body should pancake through the air in such a manner that it would hardly attain enough velocity to break through a pavement.
Now the American experts had added other features than just the research instruments. They had built an emergency cutoff into the rocket, to cut off the fuel flow in case something went wrong. As it turned out it was a most useful device—I have seen it applied in the case of Rocket No. 14. This emergency cutoff could also be used for the radio signal which would blow the war head off the body. To accomplish the "air burst", as it was dubbed, one pound of TNT was placed on each of the four upright struts of the rocket body, just below the war head. The radio signal did its duty and the four one-pound charges went off. An observer saw what happened through a powerful glass, he told that a smoke cloud enveloped the whole rocket—and then the rocket came out of the smoke cloud in one piece and crashed as if nothing had happened. And the telemetering men also reported that most of the instruments had continued broadcasting afterwards.
After this had happened once more the men of the Applied Physics Laboratory, in charge of instrumentation for Rocket No. 9 took sterner measures, consisting of a pound of nitrostarch reinforcing each pound of TNT, "fired from a central source by dual strands of primacord actuated by two No. 3 electric caps."
That separated the war head which, ironically, was never found afterwards. But the main body did pancake to the ground, looking afterwards as if it had dropped, oh say from the roof of a hundred-foot high assembly shed. From then on the air-burst method was used whenever advisable, the burst being placed at about thirty kilometers so that the dense air had a good chance to retard the pieces. (A burst at a still higher altitude would scatter them over too large an area.)
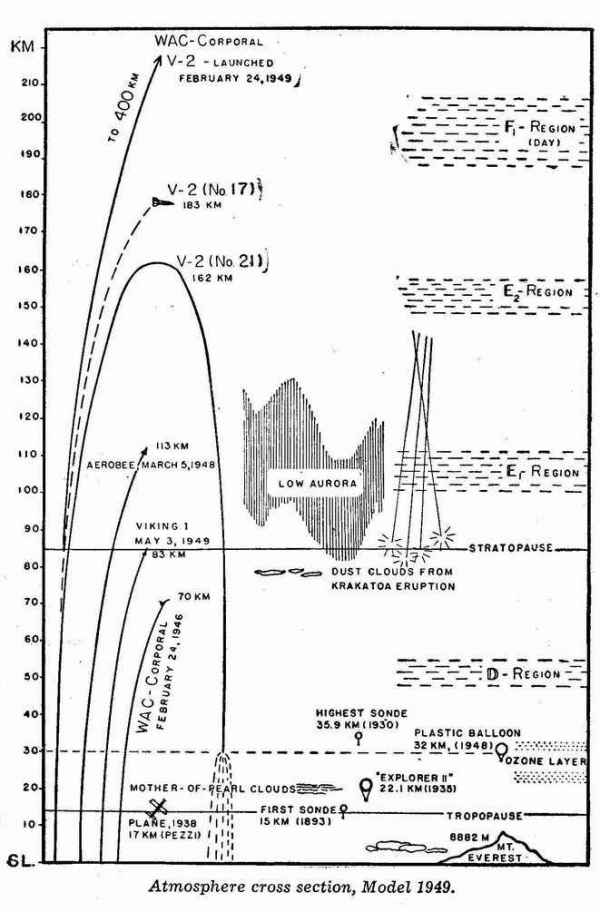
The V-2s have, as everybody knows, taken pictures of the earth, they have measured pressures and temperatures directly right up into the ionosphere. They have brought back some highly interesting information about the ozone layer formed by the sun's ultraviolet radiation at around twenty kilometers. Some indication about the existence of that ozone layer had been brought back by the Stratosphere Balloon "Explorer II" in 1935. But the balloon could enter only the sole of that layer, nobody could tell how high it would extend and any three scientists pondering that question arrived at four different answers. V-2 not only established the upper limit around 37 km—but found a double layer, separated by a layer with comparatively little ozone. On October 10, 1946 that separating layer was just at twenty kilometers, with maximum intensities at about sixteen and twenty-six kilometers.
An interesting experiment which, however, failed was first tried with Rocket No. 13. It can be readily understood that the conclusions which astronomers draw from the observations of meteors need a few assumptions about which one cannot be absolutely sure. Now if it were possible to produce a few artificial meteors about which a number of things—like particle size, probable velocity—are known one would get comparison values which will confirm or correct the assumptions. Some black powder, exploding from the large rocket which would not be disturbed much by such a small and weak explosion, might furnish some clues. Preparations were made for what was called the powder puff experiment. The engineering end of it worked, but compared to the velocity of the rocket the black powder exploded much too slowly, instead of obtaining "puffs" merely "streamers" were obtained which were of no use, except for showing that there was a high wind.
Something more powerful was needed and the shaped charge of a rifle grenade looked like the answer. Consequently Rocket No. 17, which happened to establish the altitude record for V-2s, was equipped with rifle grenades which were to fire their shaped charges at high altitudes, thus producing the equivalent of a low density meteorite. The best available information indicates that the shaped charges did not go off, the fragments of the rocket which were examined afterwards could not answer the question, since the charges may have gone off either when the air burst was made or when the war head hit the ground. Incidentally the air burst was delayed by over a hundred seconds and the device which functioned so sluggishly for this purpose was the same that was intrusted with the discharge of the grenades.
By now the condensed results of Rockets No. 1-24 are available.
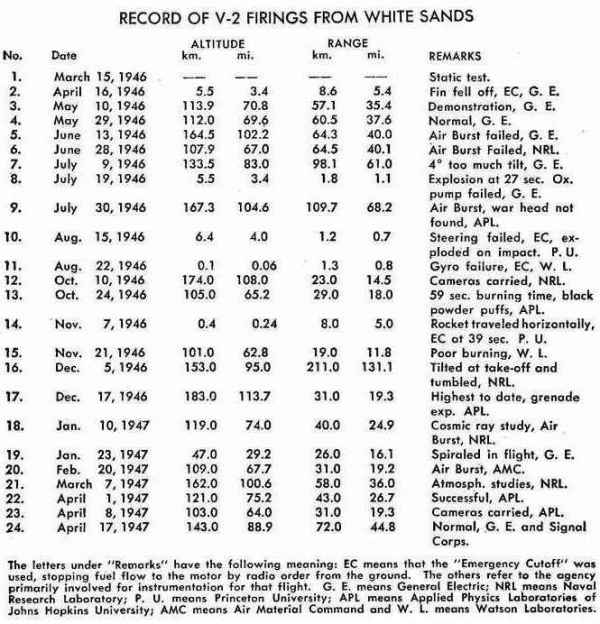
While these shots were being made, furnishing confirmation of theoretical results—such as the vertical distribution of temperature—and providing entirely new things, such as a spectrum of the sun taken from above the ozone layer and therefore far more complete than the nicest observatory spectrum, scientists began to feel that the V-2 was quite wonderful, but too big. They wanted two things, a smaller and cheaper rocket on the one hand, and a large rocket with variable payload on the other. As for the large rocket with variable payload—V-2, of course, was designed for a war head of standard weight—the Navy's Viking is the forthcoming answer. As for the smaller rocket there had first been some hopes for the WAC Corporal, but it had two drawbacks. One was that it did not quite penetrate the stratosphere, the other that it just did not have much space for instruments.
For this reason the rocket Aerobee—called also Venus temporarily before it was finished—was developed. The rocket itself is cylindrical, with a diameter of fifteen inches, equipped with three small stabilizing fins. The overall length is two hundred twenty- six inches of which eighty-eight inches represent the Instrumentation Cone, actually it is not a cone but an ogive. The Aerobee is launched by means of a solid fuel booster which imparts a velocity of some three hundred meters per second and then drops off.
The Aerobee's own motor keeps going for forty-five seconds and accelerates the rocket to a maximum velocity of about thirteen hundred meters per second, resulting in a peak altitude of about one hundred ten kilometers. The payload can be made to vary between one hundred and two hundred fifty pounds, influencing performance, of course. The first Aerobee was fired on November 24, 1947, but because of excessive tilt the emergency cutoff had to be used after thirty-five seconds. The second, fired on March 5, 1948 was fully successful and since then many Aerobecs have journeyed into the lower ionosphere, a number of them having been fired from shipboard. Just in passing it may be mentioned that a set of photographs of the earth which got into the newspapers as "pictures taken from V-2s" were actually taken from an Aerobee rocket. Since naturally not every frame of such a film is useful it so happens that the Aerobee pictures which were published were taken from a higher altitude than the corresponding V-2 pictures, even though the V-2 can go much higher than the Aerobee.
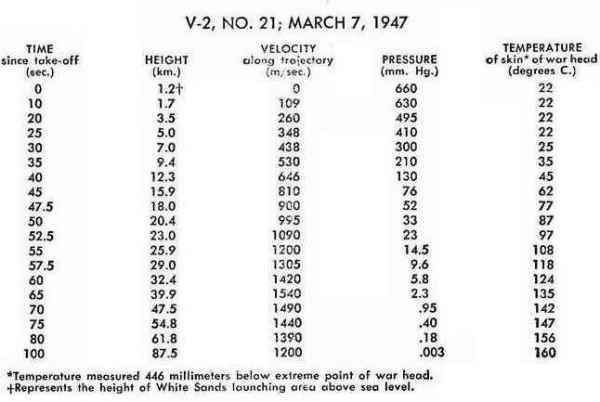
"Blazing Heat Found At High Altitudes" is the headline of a newspaper clipping I have in front of me and the reference is to a V-2 shot made on March 7, 1947-Rocket No. 21—which telemetered data to the ground from which it could be concluded that the temperature between fifty and sixty kilometers was three hundred twenty degrees Kelvin, counting from absolute zero. This makes 47° C or 116.6° F. My first guess is that the newspaper writer thought that 320° K must be at least 320° F and probably more. He probably wondered whether the rocket melted.
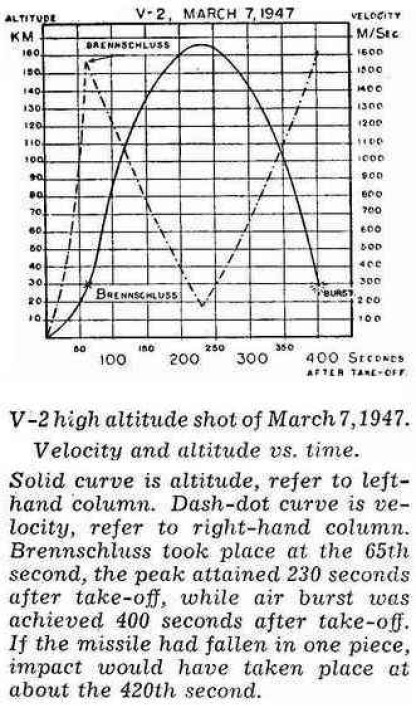
Now Rocket No. 21 happened to be devoted especially to atmosphere research. It also happened to be especially successful, everything went well and in addition to other things the temperature of the skin of the war head was measured. The problem of the heat of the rocket's skin had worried the Germans, too, when they designed it. They had measured it by putting plugs of alloys of different melting points into the skin of the rocket and looking after the test firing which alloys had melted and which had not. They found that the highest temperature at any point was around 650° C-12000 F-but they also knew that this high heating took place while the rocket was falling, entering dense layers of the atmosphere with ever increasing velocity. That the rocket would get very hot—as one uses that term when it comes to metals—while ascending was unlikely and the measurements taken at White Sands confirmed this opinion.
As regards a higher temperature in the upper stratosphere, that had been taken for granted for quite some time. It had been the explanation advanced by Professor Whipple for the "zone of silence": air of different temperature would act as a mirror for sound waves, just as the ionized layers acted as mirrors for electric waves. Temperature, it had been guessed correctly, would begin with a fairly high value at the bottom, where the ground warms the air—not the other way round—then fall off for a while, rise again, fall off some more and in the end do something which could not be predicted too well.
In 1946 things had progressed far enough so that the NACA could prepare a table of conditions from twenty kilometers to one hundred twenty kilometers, the lower ionosphere. All kinds of research, the rockets coming in only at the tail end, had indicated that the ideas of Teisserenc de Bort, and later of Wegener and Arrhenius, about the "stratified" stratosphere did not hold true. The stratosphere was not uniformly cold, as they had thought. Nor was it without winds, some meteor trails had demonstrated that conclusively. And it certainly did not show any separation of the gases, we are now satisfied that the composition of the air does not vary to any noticeable extent between bottorn and stratopause, not counting the phenomenon of the ozone layer. Of course there is much less air in a cubic mile of space fifty kilometers up, but the percentage composition is the same.
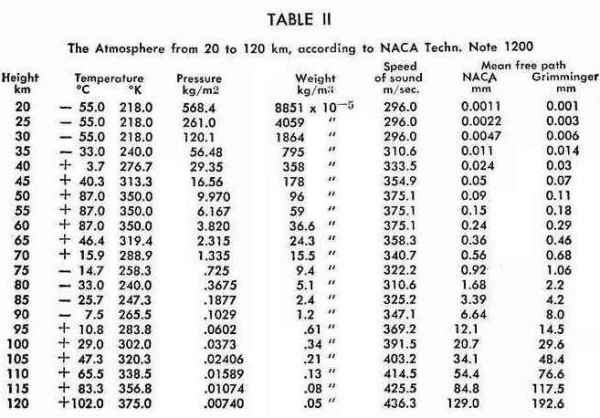
Table II gives some excerpts from the second set of NACA tables, in intervals of five kilometers. The reason why the first line in that table, for twenty kilometers altitude, does not agree perfectly with the last line in Table I is due mainly to one factor. In the first set of NACA the standard value for gravity had been used throughout, in the new set, extending to much higher altitudes, the inverse square law had to be taken into consideration. And because of the specialized questions which missile designers and their ilk were likely to ask a few things were added which had not been in the first set, speed of sound, for one.
The most interesting column, to my mind, in that table is the one which gives the "mean free path''. Everybody knows that the molecules of the air are in steady and rapid motion, a motion which increases with rising temperature. At sea level, and at standard temperature— + 15° C = 59° F—you have 2.568 X 10(19) or 25,680,000,000,000,000,000 molecules per cubic centimeter. They are all in rapid motion and, naturally, bump into each other rather frequently. Between collisions they move in a straight line and the average distance which they manage to move in between two collisions is called the "mean free path". The mean free path for standard sea level conditions has been calculated and is 9.744 X 10(-6) cm which means slightly less than one ten thousandth of a millimeter. At eighty kilometers, the altitude in which the clouds from the Krakatoa catastrophe hovered, the mean free path is close to two millimeters, at ninety kilometers it has grown to around seven millimeters—a fraction above one quarter inch—and at one hundred kilometers it has grown to between twenty and thirty millimeters, depending on how the calculation is made. In short the mean free path up there is about one inch long.
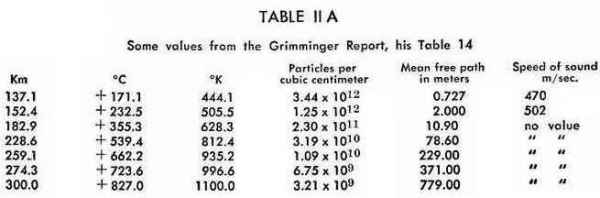
Still more recently G. Grimminger of the Rand Corporation in Santa Monica, California, has extended calculations of this kind to even higher altitudes as part of a report written for the United States Air Force. Some of his figures are given here as Table II A. The mean free path for one hundred fifty kilometers altitude works out to an even two meters, at two hundred sixty kilometers it has grown to two hundred twenty-nine meters and at three hundred kilometers to seven hundred seventy-nine meters! The number of particles per cubic centimeter for that altitude turns out to be 3,210,000,000 and that is an interesting figure. Because it was always said that a really good hard vacuum made in the laboratory will leave only a little more than 3,000,000,000 molecules per cubic centimeter. The "atmosphere" at three hundred kilometers, therefore, corresponds to about the best vacuum we can produce at the bottom of the air ocean.
Now the trouble with many of the figures in Tables II and II A is that they will be greatly misleading to many. The Aerobee rocket went to one hundred thirteen kilometers in March 1948, let's say one hundred fifteen kilometers because our abbreviated table does not contain the figures for one hundred thirteen kilometers. Ahem, so that rocket will heat up to 83.3 degrees centigrade, the speed of sound is 425.5 meters per second and if the rocket should still move with, say 850 m/sec—the muzzle velocity of a high powered rifle—its Mach number will be just 2.0. Not with a mean free path of almost one meter, my friend!
All these ideas about Mach number, speed of sound and temperature are bottom layer concepts, they all have a hidden assumption which says that the air "is a perfect gas, obeying the general thermodynamic equation of stale". It means that the air is a continuous medium. With a mean free path of a meter or so it isn't any more. Pressure has been exchanged for the impact of individual molecules. Since sound means the propagation of a pressure disturbance in air, it is no longer propagated. Consequently the "speed of sound" is now only the numerical result of a certain equation, but no longer an actuality that could be measured by two observers shouting to each other.
It is probably correct to say that at high altitudes, say from the stratopause on, sound and speed of sound no longer have a value at all, they have petered out, simply disappeared, because of the long distance between gas molecules. The problem of the shock wave is similar, but complicated by the fact that the size of the object has to enter into the picture in some manner. At an altitude where air resistance already consists of the impact of individual air molecules for a bullet moving through, it is still a semblance of a continuous medium for a V-2 rocket. And where density has degenerated to the point where a V-2 experiences the impact of separate molecules a spaceship still finds a continuous medium. Experts are not in a very perfect agreement at this moment how thin a shock wave can be, but they are agreed that it must be more than one mean free path. Some consider five to seven times the mean free path a probable thickness, others are willing to grant twice the mean free path as minimum thickness. At one hundred eighty-three kilometers, the V-2 record, the mean free path is eleven meters, it would need an enormous disturbance, a big ship, to produce shock waves which would have to be about a hundred feet in thickness.
In that altitude the value for temperature—three and a half times that of boiling water—has also lost the meaning attached to that word down here at sea level. Temperature, by definition, is the velocity of the molecules, and from that point of view the temperature at one hundred eighty-three kilometers is three hundred fifty-five degrees centigrade. But when Miss Smith moans that it is "a hundred"—meaning 100° F or 37.8° C—she is not thinking of the velocity of the air molecules. She has something in mind which falls under the classification of heat transfer, she means that a dish of ice cream, left on the desk, will first melt and then assume the temperature of 100° F. With only 2.3 X 10(11) particles around per cubic centimeter that does not apply any more. To use a comparison, not completely valid but the best I can think of, you might as well expect people in an assembly hall to die of heat because the hall is being heated by two pinheads glowing at three thousand degrees.
So we might say that at the stratopause, or somewhere in that region, temperature "disappears" too, the same as sound and speed of sound and shock waves. Physicists are beginning to talk of "kinetic temperature" under these circumstances, but the terminology has not yet settled.
In short, our surface concepts hold more or less true up to the stratopause, as does the composition of the air. From then on the surface concepts are no longer there and the composition actually begins to change. At sea level we had the customary seventy-eight percent of N(2) plus 21 percent of O(2) plus one percent of argon and rarer gases. Near the stratopause we probably have simply eighty-two percent N(2) and eighteen percent O(2). But above the stratopause we begin to get oxygen atoms, O instead of O(2). At one hundred twenty kilometers, it is believed, we have—in round figures- thirty percent O and seventy percent N(2). Whether the N(2) changes to N, too, in still higher altitudes is under discussion at the moment, but it does not seem likely.
And where is the limit of the atmosphere according to present day concepts? Well, that is, unfortunately, completely a matter of definition. At two hundred eighty kilometers we have the F(2)-Layer which reflects certain frequencies of radio waves, at least half the time, so we can definitely say that there is still "atmosphere" of some sort at two hundred eighty kilometers, since it has a property left. But above the F(2)-Layer all doors are open.
Grimminger has constructed three models, which I'll call G-I, G-II and G-III, based upon three sets of assumptions. In his first model he defines the "limit" of the atmosphere as the height where the properties of the atmosphere become the same as those of interstellar gas, better called interplanetary gas in this connection. The "kinetic temperature" of this gas is assumed to be 10,000" K., the calculation ends up with two particles per cubic centimeter at 10,657 km—6,622 miles—from the surface.
The Model G-II is based on the idea of a height h* above which there are so few molecules that a particle, starting from that height h* with escape velocity, would actually escape because it would not suffer a collision—on the average. This height h* can be calculated to be somewhere between five hundred seventeen and seven hundred twenty- two kilometers. Above h*, then, there is a region where each molecule describes a dynamical orbit in a gravitational field, the area of "molecular satellites" which Dr. Lyman Spitzer has termed the "exo-sphere". Working on this basis Grimminger found ten particles per cubic centimeter in 1,931 km—1,200 miles—altitude and less than one particle per cubic centimeter in 2,253 km—1,400 miles. Model G-III attempts to combine the assumptions of Model G-TI with the effects of diffusion equilibrium. In that model there are still twenty particles per cubic centimeter at forty thousand kilometers.
These three models are indubitably only the first groping attempts and will certainly be superseded by others. Grimminger himself quotes in his report a letter written to him by Dr. Lyman Spitzer, saying: "It is difficult to reconcile the high temperatures found for the upper atmosphere of the earth with what is known about the atmosphere of Mars ... one would suppose that the temperature of the upper atmosphere of Mars would be about the same as for the earth. At such a high temperature the carbon and oxygen atoms would have left Mars long ago, in contradiction to the observed presence of these "elements. The answer to this dilemma is certainty not clear, since the various lines of evidence pointing to a high temperature for the upper atmosphere of the earth seem rather strong. However, one cannot wholly exclude the possibility that the temperature of the earth's upper atmosphere may be considerably less than that which we have been led to assume." And there you are. It won't even help us to send a rocket halfway to the moon to photograph the earth. The result would only be what al-Biruni calculated eight hundred years ago, the pictures would show the ninety kilometers or so which have optical properties. In all probability the "exosphere" will remain a theoretical battleground for decades to come.
Not that it matters very much, for practical purposes there is nothing in the way above one hundred eighty kilometers.
References:
Upper Atmosphere Research Report No. I. Issued by Naval Research Laboratory, Report R-2955, October 1946
Upper Atmosphere Research Report No.
NRL Report R-3030. December 1945 Upper Atmosphere Research Report No.
NRL Report R-3120, April 1947 Upper Atmosphere Research Report No.
NRL Report R-3171, October 1947 High Altitude Research Using the V-2
Rocket; Bumblebee Scries Report No. 81. July 1948, Section T. A{>plied Physics Lab. (Johns Hopkins University.)
Analysis of Temperature. Pressure and Density of the Atmosphere Extending to Extreme Altitudes, by G. Grimmin- ger, November 1948. (The so-called Grimminger Report, or Rand Report.)
Centennial Symposia, December 1946. (Harvard Observatory Monographs, No. 7, published 1948.) The Atmospheres of the Earth and Planets, edited by Gerard P. Kuiper, (U. of Chicago Press, 1948.) Applications of the Theory of Free Mole- cule Flow to Aeronautics, by Holt Ashley, M. L T. (Journal of the Aeronautical Sciences, February 1949.)
Machmeters for High-Speed Flight Research, by Herbert Schaefer, (J. of the Aeronautical Sc., June 1948.)
Standard Atmosphere, 1925, reprinted 1942, N. A. C. A. Report No. 218.
Tentative Tables for the Properties of the Upper Atmosphere, N. A. C A. Techn. Note No. 1200, January 1947.
Click to join 3rdReichStudies
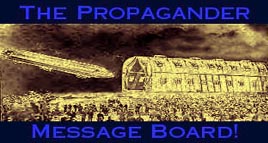
Disclaimer:The Propagander! includes diverse and controversial materials--such as excerpts from the writings of racists and anti-Semites--so that its readers can learn the nature and extent of hate and anti-Semitic discourse. It is our sincere belief that only the informed citizen can prevail over the ignorance of Racialist "thought." Far from approving these writings, The Propagander! condemns racism in all of its forms and manifestations.
Fair Use Notice: The Propagander! may contain copyrighted material the use of which has not always been specifically authorized by the copyright owner. We are making such material available in our efforts to advance understanding of historical, political, human rights, economic, democracy, scientific, environmental, and social justice issues, etc. We believe this constitutes a "fair use" of any such copyrighted material as provided for in section 107 of the US Copyright Law. In accordance with Title 17 U.S.C. Section 107, the material on this site is distributed without profit to those who have expressed a prior interest in receiving the included information for research and educational purposes. If you wish to use copyrighted material from this site for purposes of your own that go beyond 'fair use', you must obtain permission from the copyright owner.
Sources: Two superior Yahoo! Groups, magazinearchive and pulpscans, are the original sources for the scans on this page. These Groups have a number of members who generously scan and post rare and scarce magazine and pulp scans for no personal gain. They do so for love of the material and to advance appreciation for these valuable resources. These Groups are accepting new members; join now!